Physiological characteristics of the choroid and its association with myopia in children and adolescents
-
摘要: 脉络膜是位于巩膜和Bruch膜之间的一个多功能动态结构,可能参与了眼球生长调节与近视发展,脉络膜厚度可能是预测儿童青少年近视发展及治疗防控效果的重要生物标志物。研究对脉络膜的生理结构、测量方法进行了综述和总结,探讨了脉络膜的影响因素,包括年龄、生理变化、屈光状态和眼轴长度、药物影响及光学环境等,指出了脉络膜厚度在儿童青少年近视研究中可能的应用。Abstract: The choroid is a multifunctional dynamic structure located between the sclera and the Bruch membrane, which may be involved in the regulation of eye growth and the development of myopia. Choroidal thickness may serve as an important biomarker for predicting the development of myopia and the effectiveness of myopia control treatments in children and adolescents. The study reviews and summarizes the physiological structure and measuring methods of the choroid, and discusses its influencing factors including age, physiological changes, refractive status, axial length, drug effects, optical environment and so on. The review points out the potential applications of choroidal thickness in myopia research among children and adolescents.
-
Key words:
- Choroid /
- Physiology /
- Myopia /
- Influencing factor
1) 利益冲突声明 所有作者声明无利益冲突。 -
执行功能是一项认知技能,在复杂的、目标导向的行为规划、组织和监控中引发自上而下的控制过程[1]。抑制性控制、工作记忆、认知灵活性以及推理、计划和解决问题等更高层次的功能是执行功能的核心功能[2-3]。良好的执行功能对儿童青少年的社会适应[2]、睡眠时间[4]和质量[5]以及身心健康[6]都是非常必要的。
近年来,我国十分重视执行功能领域的相关研究,随着将其应用于不同领域、不同人群,开发具有针对性的评价工具成为迫切需求。之前多数研究采用实验室任务评估儿童的执行功能,近来一些研究者开始采用量表评估儿童在日常生活中表现出来的执行功能[7]。国外研究者构建了许多量表工具评估不同人群在各种认知、行为、情感领域的自我意识能力。国内学者引进和检验了国外不同人群执行功能量表,应用于评估学前儿童[8]和大学生[9-10]执行功能的可行性和有效性。
国外针对小学阶段儿童执行功能的评价量表有2008年Thorell等[11]编制的儿童执行功能量表(Childhood Executive Functioning Inventory),该量表由父母填写,有学者对其应用于学前儿童的信效度进行了检验,结果较好。2016年,Geurten等[12]编制了7~14岁儿童执行功能量表(Questionnaire of Executive Functioning,QEF),该量表是最新且最全面的,已有英文、法文等多个版本,应用于我国大学生执行功能评价的信效度得到了较高的认可[9]。本研究旨在考察该量表在我国小学生群体中的心理测量学属性,重点检验其效度和信度。
1. 对象与方法
1.1 对象
样本1:主要用于项目分析、探索性因素分析。于2022年9月5—16日,采用分层随机整群抽样法抽取研究对象,首先按市分层,采用方便抽样在广州、揭阳、韶关、茂名各选取1所小学,然后以年级分层,采用抽签法在各年级选取1~2个班级,以班级为单位纳入7~12岁学生。共发放873份问卷,去除无效问卷32份,剩余有效问卷841份,有效率为96.3%。其中,男生437名(52.0%),女生404名(48.0%);7岁学生69名,8岁90名,9岁176名,10岁188名,11岁151名,12岁167名。
样本2:主要用于验证性因子分析、效标效度检验。于2022年9月5—16日,采用方便抽样的方法从样本1所抽取的班级中选取9个班级,以班级为单位纳入7~12岁学生。共发放400份问卷,去除无效问卷23份,有效问卷377份(男生195名,女生182名),有效率为94.3%。
样本3:主要用于重测信度检验。调查时间为2022年9月22日,采用方便抽样抽取广州市某小学学生87名(男生49名,女生38名),间隔15 d后再次填写中文版QEF。
本研究调查前获得学生和家长的知情同意,并获得广州体育学院伦理委员会批准(批准号:2023LCLL-25)。
1.2 研究工具
1.2.1 7~14岁执行功能问卷
Geurten在2016年编制的QEF包含36个条目,其中涉及注意集中、工作记忆、自我调控、心理理论、灵活转换、冲动抑制、计划发动和情绪调节8个因子。原量表各个因子间的内部一致性系数为0.67~0.92,总体内部一致性系数为0.89。所有条目采用1分(从不)到4分(总是)4级评分方式。得分越高,执行功能表现越差[9]。
该问卷在经过原作者授权同意后,邀请2名心理学专业的教师和1名英语专业的研究生将其翻译成中文,由2名心理学教师对每个条目进行认真审核后,再由未阅读过本问卷的英语教师回译后,通过邮件的形式发送给原作者审核,以此确保问卷的翻译质量。
1.2.2 Rothbart儿童行为问卷
Rothbart等[13]研制的儿童行为问卷,可以用来评价儿童的自我调控能力,且在中国儿童行为中的应用具有较好的结构效度。问卷包含注意集中、注意转移、冲动性和抑制性4个因子,每个因子采用1分(从不)到5分(总是)5级评分方式。分数越高说明儿童行为的自我调控能力越好,路腾飞等[14]研究认为该量表具有较高的信效度,各因子的Cronbach α系数分别为0.80,0.70,0.74,0.76。
1.2.3 简式UPPS-P冲动行为量表(Short Version of the UPPS-P Impulsive Behavior Scale, S-UPPS-P)
本研究采用薛朝霞等[15]于2017年修订的简式S-UPPS-P冲动行为量表,该量表共有20个条目,涉及5个因子,主要包括感觉寻求、缺乏坚持性、缺乏预见性、负性急迫性、正性急迫性。该量表采用李克特4级评分:非常不符合(1分)、比较不符合(2分)、比较符合(3分)、非常符合(4分),得分越高表明个体特质的冲动性越强。本研究该量表的内部一致性信度为0.84。本文选取的S-UPPS-P冲动行为量表虽然主要用于评估冲动行为,但由冲动行为引发的情绪变化、缺乏预谋等都与执行功能所评价的维度存在一定的联系[16]。因此,认为S-UPPS-P冲动行为量表可以作为检验中文版QEF的效标量表。
1.3 质量控制
调查前,统一培训调查人员,要求其熟悉问卷的所有内容。在被调查学校的配合下,由经统一培训的调查人员集中发放并当场收回问卷,问卷填写完成后现场检查,及时补充缺失值。调查问卷回收后,统一培训数据录入员,明确录入标准。录入后对问卷数据进行严格检查,剔除规律性填答、缺漏项大于15%的问卷。
1.4 统计学方法
运用SPSS 25.0软件对数据进行录入和基本统计分析,采用独立样本t检验比较两组在各项目上的得分差异。采用AMOS 23.0软件进行探索性和验证性因素分析。采用Pearson相关分析检验量表各条目与总分之间的相关性。检验水准α=0.05。
2. 结果
2.1 项目分析
运用样本1分析各条目与分量表得分、量表总分的相关性,依据量表总分的高低顺序对受试者进行排列,得分最高和最低的27%分别组成高分组和低分组,结果显示,36个条目的决断值在14.87~27.52,两组差异有统计学意义(P < 0.01),说明量表的各条目具有较好的区分度,可以进行下一步的研究分析。Pearson相关分析结果显示,条目与量表总分的相关系数为0.22~0.46,且均有统计学意义(P值均 < 0.01)。
2.2 结构效度
2.2.1 探索性因素分析
运用样本1中的被试数据对中文版QEF进行探索性因素分析。结果显示KMO值为0.81,Barlett检验χ2为2 903.61(P < 0.01,df=190),表明适合探索性因素分析。采用主成分分析法和最大方差法,提取特征值大于1的因子,同时结合碎石图分析,并以原文献作为参考,最终确定提取8个因子,累积方差贡献率为56.68%,各项目载荷0.41~0.74。按照Geurten等的命名,将8个因子分别命名为注意集中、工作记忆、自我调控、心理理论、冲动抑制、计划发动、灵活转换和情绪调节。见表 1。
表 1 中文版QEF的因子载荷Table 1. Factor load of the Chinese version of QEF因子 条目 载荷 因子 条目 载荷 注意集中 1 0.58 自我调控 10 0.48 5 0.53 12 0.69 8 0.67 18 0.51 20 0.45 23 0.42 29 0.51 32 0.74 工作记忆 2 0.43 心理理论 3 0.69 9 0.64 4 0.71 14 0.55 16 0.52 22 0.47 24 0.63 冲动抑制 11 0.72 灵活转换 6 0.56 17 0.65 13 0.43 25 0.63 26 0.62 34 0.44 情绪调节 19 0.62 36 0.57 21 0.58 计划发动 7 0.69 30 0.41 15 0.47 31 0.70 27 0.64 33 0.54 28 0.53 35 0.52 2.2.2 验证性因素分析
根据上述探索性因素分析的结果,采用AMOS 23.0软件对样本2的问卷数据进行单因素验证性因素分析,具体结果为:χ2/df=3.048,CFI=0.988,TLI=0.980,RMSEA=0.058,SRMR=0.009,表明单因子模型拟合良好。
2.2.3 效标关联效度
表 2结果表明中文版QEF与Rothbart儿童行为问卷总分及各因子分均呈正相关(r=0.17~0.69,P值均<0.01);中文版QEF与S-UPPS-P冲动行为量表总分及各因子分均呈正相关(r=0.14~0.67,P值均<0.01)。
表 2 Rothbart儿童行为问卷及S-UPPS-P冲动行为效标量表与中文版QEF得分相关性分析(r值,n=377)Table 2. Correlation analysis of scores between the Chinese version of QEF, Rothhart Children's Behavior Questionnaire, and S-UPPS-P Impulsive Behavior Scale(r, n=377)量表 因子 注意集中 工作记忆 自我调控 心理理论 灵活转换 冲动抑制 计划发动 情绪调节 QEF总分 Rothbart 注意集中 0.69 0.62 0.58 0.43 0.67 0.40 0.55 0.61 0.63 儿童行为问卷 注意转移 0.56 0.61 0.23 0.19 0.68 0.44 0.59 0.50 0.56 冲动性 0.25 0.22 0.37 0.28 0.31 0.50 0.26 0.19 0.35 抑制性 0.47 0.53 0.24 0.17 0.56 0.60 0.63 0.50 0.52 总分 0.58 0.61 0.53 0.59 0.66 0.68 0.61 0.54 0.63 S-UPPS-P 感觉寻求 0.42 0.35 0.38 0.51 0.20 0.47 0.54 0.49 0.37 冲动行为量表 缺乏坚持性 0.18 0.14 0.22 0.17 0.25 0.43 0.58 0.36 0.51 缺乏预见性 0.35 0.27 0.32 0.26 0.15 0.33 0.40 0.26 0.48 负性急迫性 0.22 0.49 0.31 0.30 0.42 0.38 0.55 0.22 0.59 正性急迫性 0.58 0.62 0.47 0.35 0.60 0.67 0.51 0.34 0.63 总分 0.50 0.45 0.61 0.53 0.44 0.65 0.42 0.64 0.57 注: P值均 < 0.01。 2.3 信度检验
运用样本3对中文版QEF的信度进行检验,结果如表 3所示,各因子Cronbach α系数在0.65~0.80,分半信度在0.62~0.83,重测信度在0.53~0.71。
表 3 中文版QEF各分量表的内部一致性系数分半信度和重测信度(n=87)Table 3. Internal consistency coefficient, split-half reliability and retest reliability of Chinese version of QEF subscales(n=87)因子 Cronbach α系数 分半信度 重测信度 因子 Cronbach α系数 分半信度 重测信度 注意集中 0.70 0.75 0.69 冲动抑制 0.73 0.78 0.62 工作记忆 0.76 0.64 0.53 计划发动 0.74 0.67 0.58 自我调控 0.79 0.83 0.54 情绪调节 0.80 0.62 0.63 心理理论 0.65 0.77 0.71 总体 0.72 0.76 0.69 灵活转换 0.68 0.63 0.65 3. 讨论
执行功能是影响学生身心健康的重要因素之一,具备良好执行功能的人,在学习和生活中可以更好地执行计划、保持专注和维持记忆,进而有助于提质增效。中文版QEF包括36个条目,条目没有删减。本研究首先采用通过多轮翻译的方式形成中文版7~12岁儿童执行功能量表;然后,邀请小学生预填写中文版QEF,优化问卷的不足;最后,检验问卷的信度和效度。项目分析结果显示,中文版QEF的36个项目决断值在14.87~27.52,每个项目与量表总分的相关系数在0.22~0.46,且均有统计学意义,说明量表中各项目具有较高的甄别力。
效度检验结果显示,中文版QEF具有较好的结构效度,探索性因素分析提取了8个因子。8个因子的构成要素合理,每个因子包含条目数较为均匀,且各因子的载荷较高,所有条目的因子载荷均在0.40以上,累积方差贡献率为56.68%。验证性因素分析结果表明单因素模型拟合良好,各项指标均达到了检验标准。效标效度结果显示,中文版QEF与Rothbart儿童行为量表、S-UPPS-P冲动行为量表均存在正相关,表明中文版QEF能够较为客观地反映小学生执行功能水平。
信度检验结果显示,中文版QEF各分量表的内部一致性系数为0.65~0.80,分半信度为0.62~0.83,表明该量表具有较好的内部一致性。重测信度为0.53~0.71,量表呈现了较好的跨时间稳定性,同时也反映了该量表具有较好的信度,可以作为测量和评估小学生执行功能的工具。
研究存在一定的局限性:(1)样本主要来自广东省粤东、粤西、粤北和珠三角地区某座城市的一所小学,问卷是否适用于中国其他区域小学生执行功能的评价还有待进一步验证;(2)英文版问卷年龄跨度为7~14岁,因此该问卷是否适用于中国初中生群体也需要在未来的研究中进一步证实。
综上所述,可以将中文版QEF在国内7~12岁小学生中应用推广,进而更加精准地评价小学生执行功能的水平,为儿童执行功能的改善提供科学、有效的评价工具。
-
[1] NICKLA D L, WALLMAN J. The multifunctional choroid[J]. Prog Retin Eye Res, 2010, 29(2): 144-168. doi: 10.1016/j.preteyeres.2009.12.002 [2] TROILO D, SMITH E L, NICKLA D L, et al. IMI-report on experimental models of emmetropization and myopia[J]. Invest Ophthalmol Vis Sci, 2019, 60(3): M31-M88. doi: 10.1167/iovs.18-25967 [3] WALLMAN J, WILDSOET C, XU A, et al. Moving the retina: choroidal modulation of refractive state[J]. Vision Res, 1995, 35(1): 37-50. doi: 10.1016/0042-6989(94)E0049-Q [4] JIN P, ZOU H, ZHU J, et al. Choroidal and retinal thickness in children with different refractive status measured by swept-source optical coherence tomography[J]. Am J Ophthalmol, 2016, 168: 164-176. doi: 10.1016/j.ajo.2016.05.008 [5] JIN P, ZOU H, XU X, et al. Longitudinal changes in choroidal and retinal thicknesses in children with myopic shift[J]. Retina, 2019, 39(6): 1091-1099. doi: 10.1097/IAE.0000000000002090 [6] MORIYAMA M, OHNO-MATSUI K, FUTAGAMI S, et al. Morphology and long-term changes of choroidal vascular structure in highly myopic eyes with and without posterior staphyloma[J]. Ophthalmology, 2007, 114(9): 1755-1762. doi: 10.1016/j.ophtha.2006.11.034 [7] READ S A, ALONSO-CANEIRO D, VINCENT S J, et al. Longitudinal changes in choroidal thickness and eye growth in childhood[J]. Invest Ophthalmol Vis Sci, 2015, 56(5): 3103-3112. doi: 10.1167/iovs.15-16446 [8] NISHIDA Y, FUJIWARA T, IMAMURA Y, et al. Choroidal thickness and visual acuity in highly myopic eyes[J]. Retina, 2012, 32(7): 1229-1236. doi: 10.1097/IAE.0b013e318242b990 [9] WANG N K, LAI C C, CHOU C L, et al. Choroidal thickness and biometric markers for the screening of lacquer cracks in patients with high myopia[J]. PLoS One, 2013, 8(1): e53660. doi: 10.1371/journal.pone.0053660 [10] ULAGANATHAN S, READ S A, COLLINS M J, et al. Daily axial length and choroidal thickness variations in young adults: associations with light exposure and longitudinal axial length and choroid changes[J]. Exp Eye Res, 2019, 189: 107850. doi: 10.1016/j.exer.2019.107850 [11] OSTRIN L A, HARB E, NICKLA D L, et al. IMI-the dynamic choroid: new insights, challenges, and potential significance for human myopia[J]. Invest Ophthalmol Vis Sci, 2023, 64(6): 4. doi: 10.1167/iovs.64.6.4 [12] WU H, CHEN W, ZHAO F, et al. Scleral hypoxia is a target for myopia control[J]. Proc Natl Acad Sci USA, 2018, 115(30): E7091-E7100. [13] LIU Y, WANG L, XU Y, et al. The influence of the choroid on the onset and development of myopia: from perspectives of choroidal thickness and blood flow[J]. Acta Ophthalmol, 2021, 99(7): 730-738. doi: 10.1111/aos.14773 [14] PLATZL C, KASER-EICHBERGER A, BENAVENTE-PEREZ A, et al. The choroid-sclera interface: an ultrastructural study[J]. Heliyon, 2022, 8(5): e09408. doi: 10.1016/j.heliyon.2022.e09408 [15] FIELDS M A, DEL PRIORE L V, ADELMAN R A, et al. Interactions of the choroid, Bruch's membrane, retinal pigment epithelium, and neurosensory retina collaborate to form the outer blood-retinal-barrier[J]. Prog Retin Eye Res, 2020, 76: 100803. doi: 10.1016/j.preteyeres.2019.100803 [16] ALM A, BILL A. Ocular and optic nerve blood flow at normal and increased intraocular pressures in monkeys (Macaca irus): a study with radioactively labelled microspheres including flow determinations in brain and some other tissues[J]. Exp Eye Res, 1973, 15(1): 15-29. doi: 10.1016/0014-4835(73)90185-1 [17] FUJIWARA A, SHIRAGAMI C, SHIRAKATA Y, et al. Enhanced depth imaging spectral-domain optical coherence tomography of subfoveal choroidal thickness in normal Japanese eyes[J]. Jpn J Ophthalmol, 2012, 56(3): 230-235. doi: 10.1007/s10384-012-0128-5 [18] QI Y, LI L, ZHANG F. Choroidal thickness in Chinese children aged 8 to 11 years with mild and moderate myopia[J]. J Ophthalmol, 2018, 2018: 7270127. [19] DELSHAD S, COLLINS M J, READ S A, et al. The time course of the onset and recovery of axial length changes in response to imposed defocus[J]. Sci Rep, 2020, 10(1): 8322. doi: 10.1038/s41598-020-65151-5 [20] SWIATCZAK B, SCHAEFFEL F. Emmetropic, but not myopic human eyes distinguish positive defocus from calculated blur[J]. Invest Ophthalmol Vis Sci, 2021, 62(3): 14. doi: 10.1167/iovs.62.3.14 [21] KUGELMAN J, ALONSO-CANEIRO D, READ S A, et al. Automatic choroidal segmentation in OCT images using supervised deep learning methods[J]. Sci Rep, 2019, 9(1): 13298. doi: 10.1038/s41598-019-49816-4 [22] ZHANG H, YANG J, ZHOU K, et al. Automatic segmentation and visualization of choroid in OCT with knowledge infused deep learning[J]. IEEE J Biomed Health Inform, 2020, 24(12): 3408-3420. doi: 10.1109/JBHI.2020.3023144 [23] 周愉, 张敏, 朱瑜洁, 等. 深度学习在脉络膜分割中的应用研究进展[J]. 国际眼科杂志, 2023, 23(6): 1007-1011. https://www.cnki.com.cn/Article/CJFDTOTAL-GJYK202306025.htmZHOU Y, ZHANG M, ZHU Y J, et al. Research progress on the application of deep learning in choroidal segmentation[J]. Int Eye Sci, 2023, 23(6): 1007-1011. (in Chinese) https://www.cnki.com.cn/Article/CJFDTOTAL-GJYK202306025.htm [24] CHAKRABORTY R, READ S A, COLLINS M J. Monocular myopic defocus and daily changes in axial length and choroidal thickness of human eyes[J]. Exp Eye Res, 2012, 103: 47-54. doi: 10.1016/j.exer.2012.08.002 [25] HOSEINI-YAZDI H, VINCENT S J, COLLINS M J, et al. Regional alterations in human choroidal thickness in response to short-term monocular hemifield myopic defocus[J]. Ophthalmic Physiol Opt, 2019, 39(3): 172-182. doi: 10.1111/opo.12609 [26] LIM J I, FLOWER R W. Indocyanine green angiography[J]. Int Ophthalmol Clin, 1995, 35(4): 59-70. [27] SPAIDE R F, KLANCNIK J M, COONEY M J. Retinal vascular layers imaged by fluorescein angiography and optical coherence tomography angiography[J]. JAMA Ophthalmol, 2015, 133(1): 45-50. doi: 10.1001/jamaophthalmol.2014.3616 [28] WEI X, BALNE P K, MEISSNER K E, et al. Assessment of flow dynamics in retinal and choroidal microcirculation[J]. Surv Ophthalmol, 2018, 63(5): 646-664. doi: 10.1016/j.survophthal.2018.03.003 [29] IWASE T, YAMAMOTO K, RA E, et al. Diurnal variations in blood flow at optic nerve head and choroid in healthy eyes: diurnal variations in blood flow[J]. Medicine (Baltimore), 2015, 94(6): e519. doi: 10.1097/MD.0000000000000519 [30] DE CARLO T E, ROMANO A, WAHEED N K, et al. A review of optical coherence tomography angiography (OCTA)[J]. Int J Retina Vitreous, 2015, 1: 5. doi: 10.1186/s40942-015-0005-8 [31] PONGSACHAREONNONT P, SOMKIJRUNGROJ T, ASSAVAPON-GPAIBOON B, et al. Foveal and parafoveal choroidal thickness pattern measuring by swept source optical coherence tomography[J]. Eye (Lond), 2019, 33(9): 1443-1451. doi: 10.1038/s41433-019-0404-4 [32] XIONG S, HE X, ZHANG B, et al. Changes in choroidal thickness varied by age and refraction in children and adolescents: a 1-year longitudinal study[J]. Am J Ophthalmol, 2020, 213: 46-56. doi: 10.1016/j.ajo.2020.01.003 [33] LIN C Y, HUANG Y L, HSIA W P, et al. Correlation of choroidal thickness with age in healthy subjects: automatic detection and segmentation using a deep learning model[J]. Int Ophthalmol, 2022, 42(10): 3061-3070. doi: 10.1007/s10792-022-02292-8 [34] BROWN J S, FLITCROFT D I, YING G S, et al. In vivo human choroidal thickness measurements: evidence for diurnal fluctuations[J]. Invest Ophthalmol Vis Sci, 2009, 50(1): 5-12. doi: 10.1167/iovs.08-1779 [35] SAYIN N, KARA N, PEKEL G, et al. Choroidal thickness changes after dynamic exercise as measured by spectral-domain optical coherence tomography[J]. Ind J Ophthalmol, 2015, 63(5): 445-450. doi: 10.4103/0301-4738.159884 [36] OSTRIN L A, JNAWALI A, CARKEET A, et al. Twenty-four hour ocular and systemic diurnal rhythms in children[J]. Ophthalmic Physiol Opt, 2019, 39(5): 358-369. doi: 10.1111/opo.12633 [37] KIM M, KIM S S, KWON H J, et al. Association between choroidal thickness and ocular perfusion pressure in young, healthy subjects: enhanced depth imaging optical coherence tomography study[J]. Invest Ophthalmol Vis Sci, 2012, 53(12): 7710-7717. doi: 10.1167/iovs.12-10464 [38] SANSOM L T, SUTER C A, MCKIBBIN M. The association between systolic blood pressure, ocular perfusion pressure and subfoveal choroidal thickness in normal individuals[J]. Acta Ophthalmol, 2016, 94(2): e157-e158. [39] READ S A, FUSS J A, VINCENT S J, et al. Choroidal changes in human myopia: insights from optical coherence tomography imaging[J]. Clin Exp Optom, 2019, 102(3): 270-285. doi: 10.1111/cxo.12862 [40] JONAS J B, WANG Y X, DONG L, et al. Advances in myopia research anatomical findings in highly myopic eyes[J]. Eye Vis (Lond), 2020, 7: 45. doi: 10.1186/s40662-020-00210-6 [41] PROUSALI E, DASTIRIDOU A, ZIAKAS N, et al. Choroidal thickness and ocular growth in childhood[J]. Surv Ophthalmol, 2021, 66(2): 261-275. doi: 10.1016/j.survophthal.2020.06.008 [42] READ S A, COLLINS M J, VINCENT S J, et al. Choroidal thickness in myopic and nonmyopic children assessed with enhanced depth imaging optical coherence tomography[J]. Invest Ophthalmol Vis Sci, 2013, 54(12): 7578-7586. doi: 10.1167/iovs.13-12772 [43] ZHANG S, ZHANG G, ZHOU X, et al. Changes in choroidal thickness and choroidal blood perfusion in Guinea Pig Myopia[J]. Invest Ophthalmol Vis Sci, 2019, 60(8): 3074-3083. doi: 10.1167/iovs.18-26397 [44] AGAWA T, MIURA M, IKUNO Y, et al. Choroidal thickness measurement in healthy Japanese subjects by three-dimensional high-penetration optical coherence tomography[J]. Graefes Arch Clin Exp Ophthalmol, 2011, 249(10): 1485-1492. doi: 10.1007/s00417-011-1708-7 [45] HARB E, HYMAN L, GWIAZDA J, et al. Choroidal thickness profiles in myopic eyes of young adults in the correction of myopia evaluation trial cohort[J]. Am J Ophthalmol, 2015, 160(1): 62-71. doi: 10.1016/j.ajo.2015.04.018 [46] LI X Q, JEPPESEN P, LARSEN M, et al. Subfoveal choroidal thickness in 1323 children aged 11 to 12 years and association with puberty: the Copenhagen child cohort 2000 eye study[J]. Invest Ophthalmol Vis Sci, 2014, 55(1): 550-555. doi: 10.1167/iovs.13-13476 [47] TAN C S, CHEONG K X, LIM L W, et al. Topographic variation of choroidal and retinal thicknesses at the macula in healthy adults[J]. Br J Ophthalmol, 2014, 98(3): 339-344. doi: 10.1136/bjophthalmol-2013-304000 [48] LI W, JIANG R, ZHU Y, et al. Effect of 0.01% atropine eye drops on choroidal thickness in myopic children[J]. J Fr Ophtalmol, 2020, 43(9): 862-868. doi: 10.1016/j.jfo.2020.04.023 [49] YE L, SHI Y, YIN Y, et al. Effects of atropine treatment on choroidal thickness in myopic children[J]. Invest Ophthalmol Vis Sci, 2020, 61(14): 15. doi: 10.1167/iovs.61.14.15 [50] OGAWA M, TORⅡ H, YOTSUKURA E, et al. Intensive outdoor activity for 1 week increased choroidal thickness[J]. Invest Ophthalmol Vis Sci, 2022, 63(7): 246-A0100. [51] 翟露露, 伍晓艳, 许韶君, 等. 接触阳光与儿童近视关联的研究进展[J]. 中华流行病学杂志, 2016, 37(11): 1555-1560. doi: 10.3760/cma.j.issn.0254-6450.2016.11.023ZHAI L L, WU X Y, XU S J, et al. Progress in research of association between myopia and sunlight exposure in children[J]. Chin J Epidemiol, 2016, 37(11): 1555-1560. (in Chinese) doi: 10.3760/cma.j.issn.0254-6450.2016.11.023 [52] WU P C, CHEN C T, LIN K K, et al. Myopia prevention and outdoor light intensity in a school-based cluster randomized trial[J]. Ophthalmology, 2018, 125(8): 1239-1250. doi: 10.1016/j.ophtha.2017.12.011 [53] XIONG F, MAO T, LIAO H, et al. Orthokeratology and low-intensity laser therapy for slowing the progression of myopia in children[J]. Biomed Res Int, 2021, 2021: 8915867. [54] LAN W, FELDKAEMPER M, SCHAEFFEL F. Bright light induces choroidal thickening in chickens[J]. Optom Vis Sci, 2013, 90(11): 1199-1206. doi: 10.1097/OPX.0000000000000074 [55] LOU L, OSTRIN L A. Effects of narrowband light on choroidal thickness and the pupil[J]. Invest Ophthalmol Vis Sci, 2020, 61(10): 40. doi: 10.1167/iovs.61.10.40 [56] LI Z, HU Y, CUI D, et al. Change in subfoveal choroidal thickness secondary to orthokeratology and its cessation: a predictor for the change in axial length[J]. Acta Ophthalmol, 2019, 97(3): e454-e459. [57] WOLFFSOHN J S, KOLLBAUM P S, BERNTSEN D A, et al. IMI-clinical myopia control trials and instrumentation report[J]. Invest Ophthalmol Vis Sci, 2019, 60(3): M132-M160. 期刊类型引用(1)
1. 侯耀奇,王燕,邓佳琪,宋湘勤. 运动干预改善孤独症谱系障碍儿童青少年抑制控制功能的研究进展. 中国儿童保健杂志. 2025(01): 92-96+102 . 百度学术
其他类型引用(0)
-
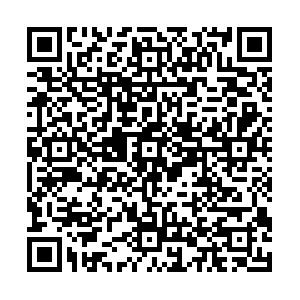
计量
- 文章访问数: 263
- HTML全文浏览量: 187
- PDF下载量: 47
- 被引次数: 1